Cells perform a complex feat of picking and proofreading amino acids for protein synthesis. Several aspects of this translation process were shrouded in mystery for a long time. This article traverses Rajan Sankaranarayanan’s two decades of seminal research that decoded some of the checkpoints and helped biologists better understand the error-free protein translation mechanisms.
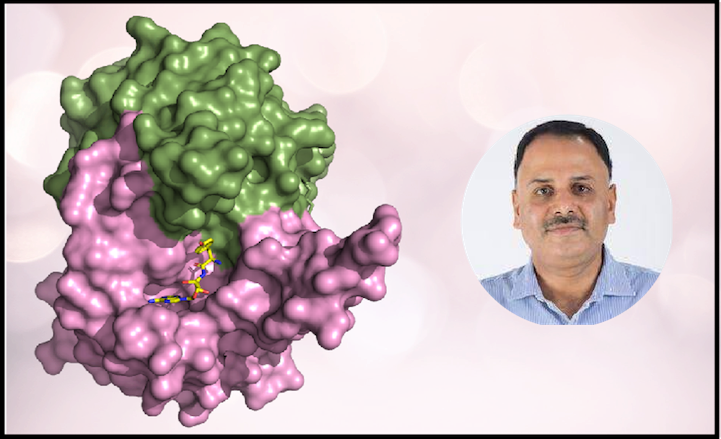
Proteins are the workhorses of living cells, and genes determine how these proteins are faithfully manufactured. Fundamentally, a protein is a string of amino acids (simple organic molecules) arranged in a specific sequence that decides the structure and function of the protein. During protein translation — the process of making proteins — a cell chooses amino acids from a set of twenty chemically distinct ones. Each of them is carried by a specific adaptor molecule called tRNA. Enzymes aid the amino acids in binding to their respective tRNAs. They are then taken to the protein translation site, where they are picked and incorporated into a new protein.
Sometimes, cells pick the wrong amino acids, as many have only minor chemical differences. But cells also have many proofreading enzymes to correct the mistakes, and such editing abilities have been key to the survival of organisms over millennia. Scientists are actively researching the details of these correction processes.
Rajan Sankaranarayanan, Outstanding Scientist, CSIR-Centre for Cellular and Molecular Biology (CCMB), Hyderabad, is one of them. As a structural biologist, he studies the structures of enzymes down to their atomic detail and correlates them with their functions in cells. Over the last two decades, he has delved deep into the proofreading and editing enzymes involved in protein translation in bacteria, archaea (the oldest unicellular life forms on earth) and eukaryotes (uni-or- multicellular organisms, evolved from the union of bacterial and archaeal cells).
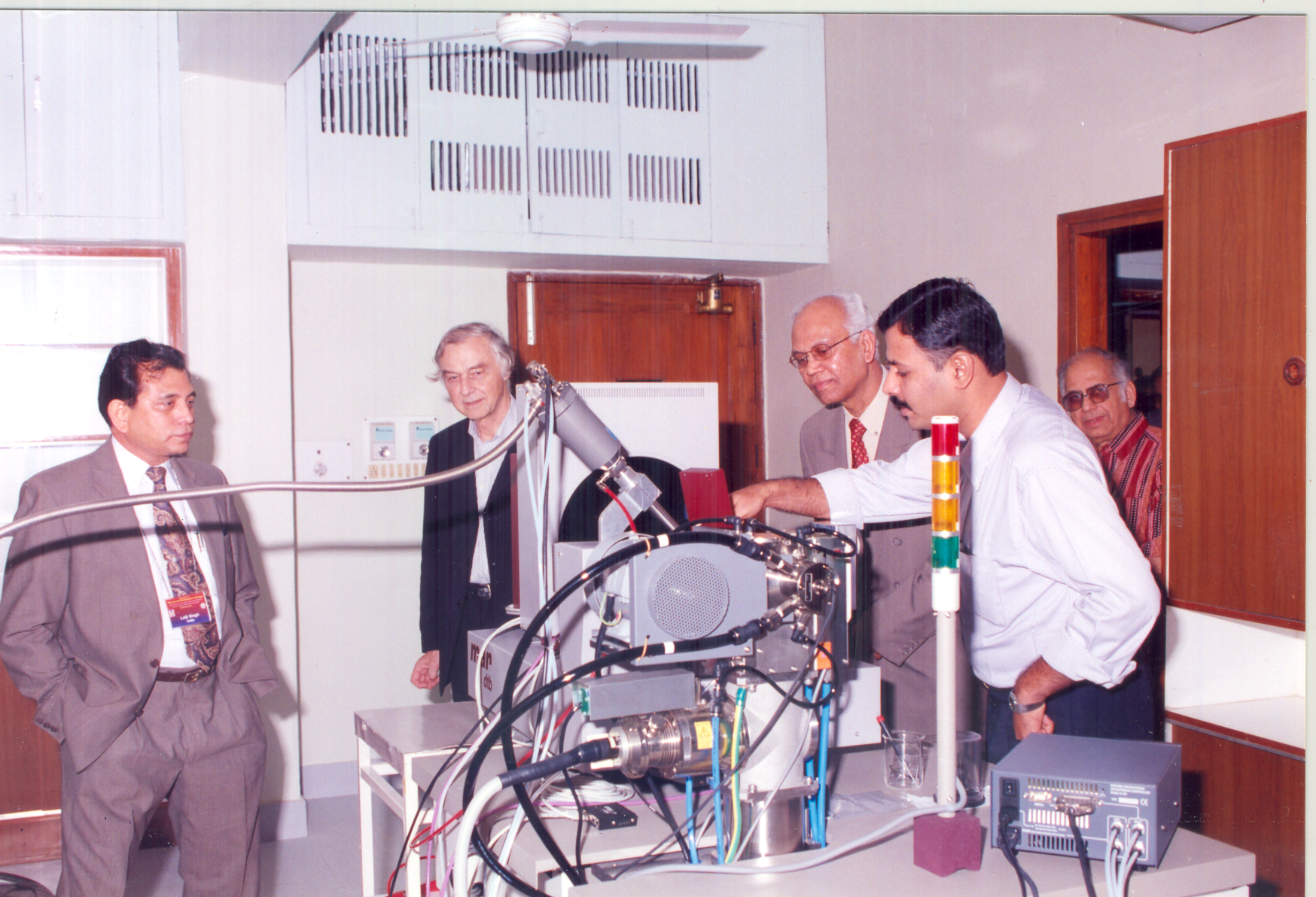
Sankaranarayanan’s exhaustive work has unravelled a few mysteries of a class of enzymes that play a vital role in the error-free translation of proteins, advancing our knowledge of these life-controlling mechanisms.
Here is a brief account of his research journey.
A serendipitous discovery leads to a deep dive
In the early 2000s, Sankaranarayanan discovered that one of the protein translation enzymes in bacteria performed a dual role: it attached amino acids to their tRNA and corrected the wrong ones (if any). In addition, he found a specific region, called an editing domain, on the enzyme’s molecular structure that was performing the editing function.
Sankaranarayanan wanted to understand this novel editing mechanism more, so he moved to CCMB in 2002 to start his research group. First, he set up a state-of-the-art X‑ray diffraction facility there. Then, the group began making crystals of various protein translation and editing molecules and studying their structures.
When studying a protein translation enzyme in archaea, they found that this enzyme, too, had an editing domain just like in the bacterial enzyme discovered earlier. But the structures of the two were different. Excited, they searched for other enzymes across organisms that might have a structurally similar domain. If they found one, it would give them a clue about the domain’s function.
The work took an unexpected turn in 2005 when they found it to be similar to another editing enzyme, called the D‑aminoacyl-tRNA Deacylase (DTD) in bacteria.
The master selector
Until then, DTD — a poorly-understood enzyme — was known to be only an amino acid chirality selector. Chirality is a structural feature of amino acids, where the amino acids are found in two mirror-image-like forms, L (for levo) and D (for dextro). Chemically, L and D chiral forms of amino acids are the same, but their 3D molecular arrangements are mirror images of each other. (Just like the structure of our palms). Nineteen amino acids exist in L and D forms except for glycine.
However, not much experimental evidence of DTD’s functions existed, and scientists were unaware that D‑amino acids were present widely inside cells. Over time, reports of D‑amino acids’ role as neurotransmitters and hormone regulators came; they were also components of bacterial cell walls and antibiotics, making it clear that cells had copious amounts of D‑amino acids but proteins do not contain them. It was evident that cells were fishing out L‑amino acids and then incorporating them into proteins.
Sankaranarayanan thought the likeness in editing domains (in bacterial and archaeal enzymes) hinted that, different organisms used similar mechanisms to remove D‑amino acids from tRNA. But the actual enzyme action was in the dark. So, he and his team started looking at crystals of DTD and D‑amino acid structures bound to tRNA.
After screening hundreds of crystals for structure determination, they realized how the two interact.
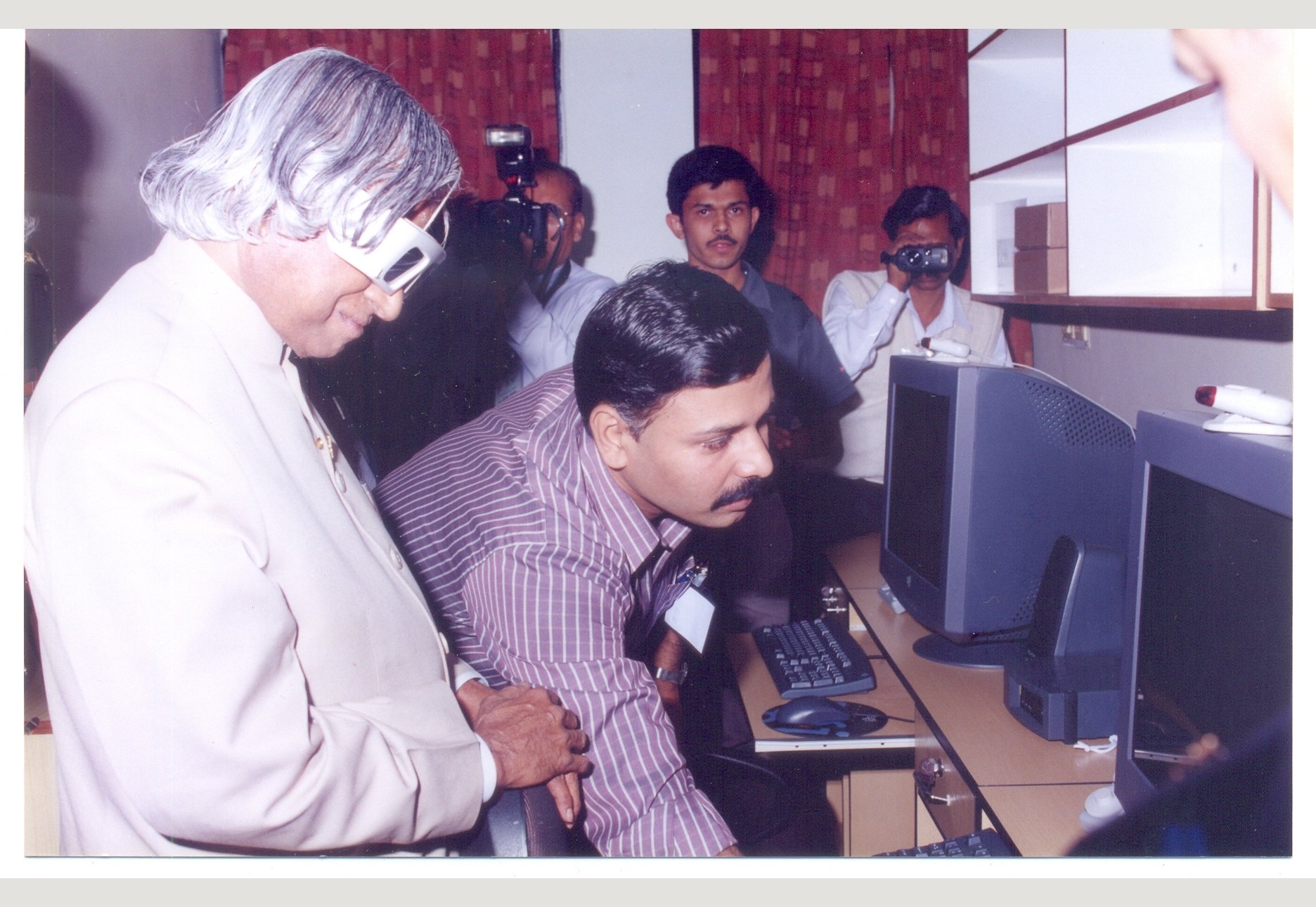
A cleft in the structure of DTD fitted into a matching structure in D‑amino acid bound to tRNA. When the two latched, a chemical reaction separated the D‑amino acid from the rest of the complex. In contrast, the complex with L‑amino acids has a structure that does not fit into the cleft and hence gets selected for protein making. For glycine (the amino acid without chirality), tRNA enters the DTD cleft but stays intact.
DTD’s fine ability to distinguish between glycine’s tRNA and other amino acids comes from identifying one position on the tRNA – called the discriminator base. However, in glycine-tRNA, the discriminator base is structurally different from all other tRNAs and hence, has a separate identity. Thus, Sankaranarayanan deciphered the mechanistic details of how DTD selects one of the mirror images for protein translation in cells.
“There are other chiral checkpoints too in the protein translation machinery in a cell. But DTD is unique because it selectively rejects binding to L‑amino acids; all others known choose binding to L‑amino acids,” says Sankaranarayanan.
By now, it was also evident that every living organism has DTD enzymes. Although they differ structurally, their function is common — to remove D‑amino acids from the selection. Sankaranarayanan’s team saw enough examples to infer that DTD and tRNA have co-evolved in unexpected scenarios.
The molecule’s multiple roles in the evolution of life
They found that DTD in eukaryotic organisms evolved from bacterial DTD. The difference lay in their choice of discriminator base on glycine tRNAs. This difference allows the modified bacterial DTD to work with archaeal tRNA. This change in DTD was necessary for protein synthesis in eukaryotic cells formed from the symbiosis of bacteria and archaea.
As the eukaryotes evolved into multicellular organisms, they noted the chances of mismatch were more between amino acids and tRNAs. Also, they found that ATD, an animal-specific DTD-like enzyme, minimized such mistakes. “Evolution of ATD and the start of multicellularity happened around the same time. So, it looks like ATD helped organisms overcome the challenges in protein translation caused by multicellularity,” says Sankaranarayanan.
In plants, the team found another enzyme called DTD2 (which had a different structure). It played the part of DTD, helping the plant roots survive underground in low-oxygen conditions by removing the detrimental effects of acetaldehyde in root cells. Acetaldehyde disrupts protein translation machinery’s function.
Sankaranarayanan’s studies compelled biologists to think of the role of DTD enzymes in the broader context of evolution. “tRNA is considered important in the evolution of life because it can pick amino acids and build proteins. Our studies now show DTD enzymes have worked with tRNA in key evolutionary steps – for the success of eukaryotes, multicellular organisms and land plants – a feat not known for any other enzyme,” remarks Sankaranarayanan.
LS Shashidhara, Distinguished Professor of Biology and Dean of Research at Ashoka University, Delhi NCR, says: “Sankaranarayanan’s work in protein quality control, with a special focus on chiral selection, is elegant, pathbreaking and qualifies to enter classical textbooks on foundations of biology.”