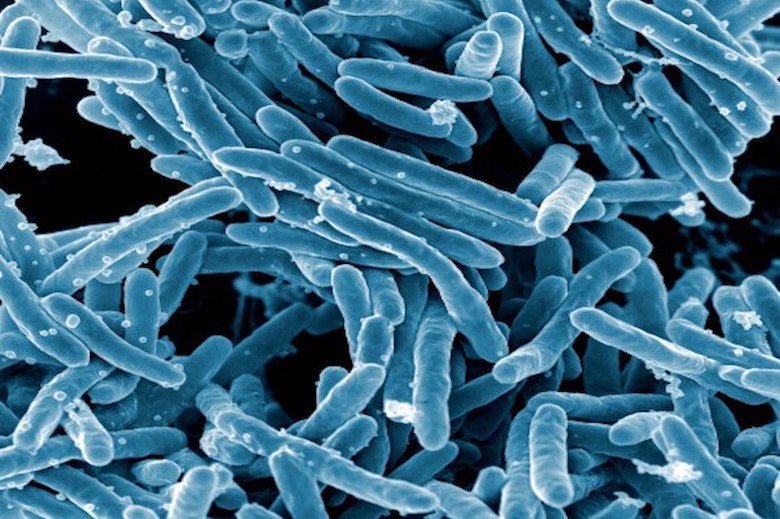
From snowflakes to spiral galaxies, many objects are “fractal” in nature. Like Russian nesting dolls, they are made up of repeating patterns that look the same at any magnification. Scientists have also noticed repeating patterns in biological phenomena such as fluctuations in heart rate or formation of bacterial colonies. Some believe that changes in DNA sequences over time also show measurable patterns, and have tried to quantify these patterns using graphical plots and mathematical parameters.
By computing such plots and parameters, a new study has found a way to distinguish between various drug-resistant varieties of tuberculosis bacteria. DNA sequences of three different varieties gave rise to distinct fractal plots and showed differences in values of defined parameters, providing potential signatures to identify each variety. The paper was published in Scientific Reports.
The evolution of drug resistance in tuberculosis bacteria is a major public health concern. Apart from a drug-sensitive (DS) variety, there are MDR (multi-drug resistant) strains that are immune to at least two powerful drugs, and XDR (extremely drug resistant) strains immune to at least four. Changes that arise in the bacterium’s DNA as it evolves are largely restricted to specific genes and certain “hotspots”.
In the current study, for each variety, the researchers took 40 strains, picked portions of DNA containing these hotspots and genes, and strung them together to generate long sequences for analysis. Then they created a graphical plot called a “DNA walk” by following a simple rule: the “walker” moves up when they come across the letter C or T, and down when the letter is A or G. Each bacterial variety gave rise to a unique fractal plot.
The researchers also calculated some defined parameters for each sequence. A parameter called correlation function, for example, measures the likelihood of finding a certain letter at a specific distance in the DNA, and obeys a mathematical rule called power law. As the gene structure undergoes changes, the exponent of the correlation function also changes, and therefore showed different values for DS, MDR and XDR.
The researchers also found that XDR showed higher values than DS and MDR varieties for three different parameters denoting genetic complexity, due to a change in its fractal nature induced by changes in its DNA.
“This type of analysis can give important insights of the genome probably at the functional level which could help clinicians or drug design,” says senior author Brojen Singh, Associate Professor, School of Computational and Integrative Sciences, Jawaharlal Nehru University.
“The findings may be significant if these results are useful in practice,” says Somdatta Sinha, Professor, Department of Biological Sciences, IISER Mohali, who was not involved with the research. The authors have shown a “mechanistic way” to differentiate between drug-resistant varieties, “thereby giving a technique to predict (hopefully) from the genome sequence of the isolate if the particular patient will show any of the above drug resistances,” she says. Whether it will still work if one takes the DNA sample from a single isolate that is small is another question, she points out, as the method requires long DNA sequences.
Sinha and colleagues previously used multi-fractal analysis to visualise distribution patterns of DNA sequences in different HIV‑1 strains. Although many studies have uncovered fractal properties in biological systems, the implications for evolutionary, developmental and molecular processes are not yet clear, she says. “That does not mean that there is no meaning; it is just that we are not able to comprehend it at this time.”